Electric vehicles are beginning to win considerable attention but are still rarely sighted on American roads. Through the first half of 2017, fewer than 800,000 battery EVs (BEVs) had been sold in the United States, or about 1 percent of all cars.1 But growth has been strong of late due to rising consumer acceptance, improved technology, and supportive regulation. McKinsey estimates that there could be ten to eleven million BEVs on US roads by 2030.2
For this to happen, though, access to charging infrastructure must improve. Although many BEVs are charged at home, public charging is necessary for owners who are travelling or if they don’t own homes with garages. Right now, there are only about 16,000 public charging stations3 in the United States; there are seven times as many gas stations. Fewer than 2,000 of these are fast charging stations because those are expensive and currently unprofitable with too few transactions to break even.4
It is a classic chicken-or-egg situation. People will be reluctant to buy a BEV if they worry that it will run out of juice. But unless more BEVs are sold, the charging infrastructure will not be built to serve them.
Two problems, one solution
There are two major problems.
First, there is convenience. Most public charging stations today are “Level 2,” meaning that they deliver 7 to 19 kilowatt-hours (kWhs) of energy every hour (think of kWhs as equivalent to gallons of gas).5 A BEV sedan with a 60-kWh battery would take five to ten hours to “fill up” at a conventional (as opposed to fast-charging) Level 2 station. Having so few stations and such long service times turns off would-be buyers (Exhibit 1).
Second, there are the economics. Although direct-current fast-charging (DCFC) stations with 150 kilowatts of power can fill up a BEV sedan in about 30 minutes, they can cost up to $150,000 to install; a 50-kilowatt DCFC station can cost $50,000. The kilowatt number refers to the maximum amount of energy that can be drawn every hour; a higher kilowatt delivers more electricity faster. DCFC stations are also expensive to run.
One reason behind the expense is “demand charge” (see sidebar, “What are demand charges?”). All electricity customers pay for the energy they consume, as measured in kWh; this charge is like paying for gallons of water used. Nonresidential customers, including charging stations, also pay a demand charge for the maximum amount of energy used in any 15- to-30-minute period in a month. Measured in kilowatts (kW), a unit of power, this charge is like paying for overhead. It is assessed to recoup the fixed costs for power plants, power lines, transformers, and so on that connect customers to the grid and supply power even at times of high demand. Demand charges account for a significant fraction of consumers’ electric bills and can make EV-charging stations unprofitable (Exhibit 2).
In the specific case of BEV charging, as soon as a car plugs in, the station owner must pay a demand charge. This is based on several factors, including the number of chargers on the site, the maximum power in kilowatts used by the car when it plugs in, and the number of cars charging at the same time in any 15- to 30-minute segment.
Would you like to learn more about the McKinsey Center for Future Mobility?
Here is a hypothetical situation. A DCFC station has four 150-kilowatt chargers. In an average month, two or three cars a day show up to charge, none at the same time. Each car uses energy at a rate of 150 kilowatts and charges for at least 15 minutes; the peak is therefore 150 kilowatts for that month. If two cars showed up during the same 15 minutes, though, the peak energy used would be 300 kilowatts, which would double the demand charge for the month.
Demand charges can be as little as $2 per kilowatt all the way to $90 per kilowatt6 ; paradoxically, they tend to be higher in states where BEVs are more popular, such as California, Massachusetts, and New York. In a high-charge state, with no cars charging at the same time, the monthly demand charge could be $3,000 to $4,500. For the BEV owner, that could translate into $30 to $50 per session, plus the cost of the actual energy. Customers just will not pay that. Clearly, if there were more customers, the cost per session would fall. But because current costs are so high, investors have been slow to build stations, and because there are not enough charging stations, consumers have been slow to buy BEVs.
There is a way to resolve this conundrum: stationary battery storage (Exhibit 3). On-site batteries can charge and discharge using direct current (DC) and connect to the grid through a large inverter. They can then charge from the grid at times when costs are lower, store the power, and release it when demand is higher (a practice known as peak shaving). When a car arrives, the battery can deliver electricity at 150 kilowatts without drawing power from the grid. If two vehicles arrive, one can get power from the battery and the other from the grid. In either case, the economics improve because the cost of both the electricity itself and the demand charges are greatly reduced.
In addition, the costs of batteries are decreasing, from $1,000 per kWh in 2010 to $230 per kWh in 2016, according to McKinsey research.7 So are the costs of the rest of the system, such as the inverter, container, software and controls, site design, construction, and connection to the grid.
Here is how it could work. A station owner installs a battery system capable of charging and discharging at a power of 150 kilowatts and builds in 300 kWh of battery cells to hold the energy. When no vehicles are present, the battery system charges up to ensure that energy is available and does not trigger a higher demand charge. When a car arrives, the stationary battery delivers the needed juice without calling on the grid. When two vehicles come in, the battery could provide power to one and the grid could provide power to the other.
A battery with a 300-kWh capacity can manage the peak demand through several two-vehicle charges and recharge in between, thus keeping peak demand below 150 kilowatts. A system configured this way could reduce demand charges to a minimum; that would be $3,000 a month that would not need to be passed on to consumers, which would substantially cut costs. Tesla has already said it is going in this direction, and others may “follow suit.”8
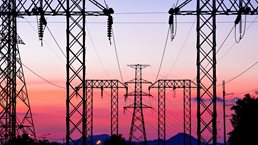
Battery storage: The next disruptive technology in the power sector
When and if BEVs hit the roads in high numbers, batteries will no longer be able to reduce peak demand efficiently because there will not be enough time to recharge them as cars queue up for power. At this point, though, economies of scale will kick in, and the demand charge will be absorbed by the many cars coming through the station.
That does not mean that on-site batteries will become obsolete. They can still be a source of value. Where costs vary widely by the hour, such as in California, batteries can reduce the per-kilowatt-hour cost of electricity. They can also generate revenue by providing additional grid services such as frequency regulation and demand response.
There is considerable optimism about EVs, and for good reason, given rising concerns about the environment, volatility in oil prices, and falling costs. McKinsey estimates that EVs, which now account for less than 1 percent of the global fleet, could hit 20 percent by 2030 (for cars) and 12 percent (for commercial vehicles).
But these are hypothetical scenarios. In reality, it is consumers who will ultimately decide the destiny of EVs. Accustomed to the ease of conventional cars, they want the same from EVs. For that to happen, charging must become cheaper and easier. By helping cut operating costs, enhance revenues, and improve reliability, battery storage could play a crucial role in this evolution.