Electrifying heat production is not just good for the planet; it is also technically feasible and increasingly cost competitive. However, enduring perceptions of industrial heat as “difficult to electrify” have hindered progress toward the decarbonization of heat.
Electrification can be an accessible and viable option to decarbonize most low- and medium-temperature heat needs across multiple sectors, including food and beverages, manufacturing, and chemicals. High-temperature heat electrification is also ongoing, as paradigm-shifting new designs and concepts are applied across industries.
As the economic feasibility of electrification improves, industrial companies have an opportunity to decarbonize and capture value—potentially with payback possible within a year—and increase resilience if they combine electrification with renewables. An integrated approach could be key to unlocking this opportunity.
In this article, we address the perceived barriers to electrification and highlight how companies can capture the growing potential for cost-effective, sustainable, and resilient heat systems.
Industrial heat: Responsible for about a fifth of global energy demand and perceived as hard to electrify
Heat (comprising industrial and building heat) is responsible for around half of global energy demand. Industrial heat is responsible for a fifth of global energy demand, accounting for a significant proportion of energy-related carbon emissions (Exhibit 1).
While electrification offers a clear pathway to decarbonization and is feasible in almost all heat categories, companies have been slow to make the transition due to long-standing concerns about technical and economic feasibility.1 A lack of knowledge on commercially available technologies for low-carbon industrial heating, such as thermal storage and heat pumps, has caused hesitation, which is amplified by the complexity and heterogeneity of industrial processes. A better grasp of commercially available technologies could help industrial companies make progress on electrification.
Companies may also lack an understanding of the changing economics of electrification. Historically, fossil fuels have been the cheapest energy source for heat production. That heat was in turn used to produce electricity (for example, in coal power plants), and part of it was lost due to the conversion efficiency. This meant that electricity generated from heat was always more expensive than the heat itself.
Additionally, in some markets, such as Europe, the cost of heat is climbing as industry must pay for CO2 emissions.2 Following the proliferation of renewable energy sources (RES), industrials have the chance to decouple the electricity price from fossil fuel prices, enabling greater cost efficiency.
However, to capture the opportunity of clean, intermittent power, industrial companies need to understand price forecasting: what are the forecasted trends and how can industrials adapt to benefit? As it stands, not all companies may have the capabilities to develop credible, hourly cost-forecast models for longer-term simulation. Even with up-to-date knowledge of pricing, they may be unfamiliar with multiple price bidding or have limited access to such contracts. Improving industrials’ forecasting capabilities could be a key lever to enable electrification.
Further, the current approach to grid costs—where supply exactly meets demand when required and energy delivery is costly—is not congruent with the variable supply from RES.3 When electricity from RES is in excess, users still pay for costly energy delivery. A new grid mechanism that can adapt to an electricity surplus is needed to set the transmission price. Such a mechanism can be more granular and, for example, introduce dynamic bidding split by congestion zone.
Electrification can also be limited by a paucity of operational incentives. The principle of “if it isn't broken, don't fix it” is deeply ingrained in engineers and, without strong incentives, companies may hesitate to change well-established processes that have proven to be reliable over many years.
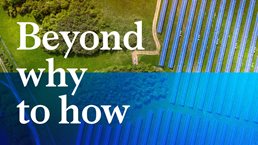
Move from plans to progress.
Concerns of technical feasibility no longer hold true
Contrary to what many companies believe, however, our analysis suggests that electrification technologies are commercially available for most low- and medium-temperature heat needs.4 The redesign of heat processes that were intended for fossil fuels can often bring additional benefits, such as a reduction of operational expenses in the long term for certain industries.
Our analysis shows that electrification is an accessible and viable option within certain conditions, with some small exceptions (see sidebar, “Our analysis”). Electrification can be undertaken today for many low- and medium-temperature heat processes (up to 600°C), but technologies for high- and very-high-temperature heat needs (above 600°C) still need to mature. This specifically refers to the transfer of heat from the electrical heating element to the product, as high temperature heating means are mature and available today.
- Low- and medium-temperature heat sectors, including food and beverages, manufacturing, and chemicals, can decarbonize using existing electrification technologies (Exhibit 2). Many industrial processes such as pasteurization, sterilization, drying, or heating require temperatures well below 600°C. An example in the food industry is milk powder production—this requires temperatures of up to 250°C and can therefore use electric solutions such as hot air heaters, superheated steam drying, and electric steam boilers.5
- High-temperature heat sectors have solutions that vary in maturity. At a small scale, industrials often use existing electrification technologies, with industrials in some countries adopting electrification technologies early due to expensive and limited liquid fuels and a focus on small-scale entrepreneurship. In glass and glass wool processes, electrification is relatively advanced, but many industries require further development. Additionally, radiant-based heating is often used for high-temperature heat—raising the importance of rethinking old furnace designs rather than replicating designs with electrical elements.
- Very high-temperature heat sectors, like cement production (which requires temperatures of up to 1,500°C), are available but at an early stage of maturity. New designs, such as Leilac, are well-suited to high-temperature heat processes as they split calcination and clinkerization, with the calcination designed for fuel arbitrage and pure CO2 production.6 According to our analysis, retrofitting is expected to have a similar capital expenditure to amine-based carbon capture, with partial electrification also possible. In the alumina production process (where temperatures up to 1,000°C are required), electrifying the calciner can enable steam recovery, which is not achievable in the current fossil fuel-based design. That steam can be used elsewhere in the plant—for example, in the bauxite digestion process—and effectively reduce the overall plant energy demand.
However, electrification is not a “one size fits all” lever for decarbonization. Bespoke solutions involving various technologies (like biofuels, geothermal, and nuclear) may be more suitable for processes and sectors across different contexts. For industries such as ethylene production, electrical crackers are being conceived and tested, with some—including new rotating equipment—showing improved yield, reduced size, and strong economic incentives.7 In high temperature processes where high energy density is needed, electrification can be combined with fuel (for example, hydrogen). Further, electricity can be used to preheat air and effectively reduce fuel demand.
Economic feasibility is improving and net-present-value-positive cases present new opportunities
The economics of decarbonizing heat via electrification are increasingly viable. First, electrification can bring additional benefits such as efficiency gains in the case of heat pumps or even better product yields (for example, in electrified ethylene production).8
Second, electricity is already often cheaper than gas. On one hand, the on-site costs of RES are going down and, on the other hand, grid electricity prices are becoming lower in moments of oversupply.9 Often, these two sources of electricity (on-site RES and grid) can be combined, leading to even lower energy costs. Third, CO2 prices are effectively making fossil fuels more expensive.
All these elements together make a good case for considering heat electrification in the industrial context. But, regardless of these overall trends, companies may still need to identify the best way to capture value from decarbonizing heat. Electricity combined with thermal energy storage is expected to have a high potential for several reasons.10 For one, electric heating can be installed quickly and in parallel to existing heating; for example, using steam, resistive liquid heating, and hot air for drying. In addition, thermal storage can be combined with both grid storage and low-cost, behind-the-meter generation.
The economic potential of electricity combined with thermal storage is supported by the decreasing costs of renewable energy generation. As more renewable electricity capacity becomes available on the grid, prices typically become more volatile. This can create arbitrage opportunities to alleviate grid bottlenecks and stabilize prices.
Another economical advantage of electrification is that the cost of electricity is becoming lower than the cost of gas and CO2 (Exhibit 3). Drops in prices generally coincide with an oversupply of RES—this means that “cheap hours” of electricity availability are also “low-emission hours” (see sidebar, “The advantages of oversizing electricity generation”).
While the large price swings of 2022 are no longer present, when we look, for example, at Germany and Spain, and consider the price spread between gas and electricity, it becomes apparent that price volatility remains (Exhibit 4). In both examples, the share of hours when electricity was cheaper than gas was around 15 to 25 percent in 2023. Price volatility may increase in the future as additional RES are added to the system.
Under the right conditions, electrification could pay back within a year
Electrification presents a swift payback time. Our analysis modeled the hourly system operations for Germany and Spain in 2021, 2022, and 2023 to illustrate examples with contexts of historical, (2021) extreme (2022), and current (2023) volatility (Exhibit 5). The model applies a hybrid system with a parallel electric boiler—a solution that can be applied most easily across industries. This system demonstrated a payback turnaround time of around eight months to five-and-a-half years, based on low capital expenditure (€125,000 per megawatt thermal [MWth]).
Our analysis also considered the increasing role of thermal systems for storing excess energy during periods of volatility. In the modeled cases, we observed payback times of just over one-and-a-half years to over four-and-a-half years. More important, over half of the energy was delivered electrically—effectively through excess “clean” electrons. However, the analysis considered that the end user would only pay an additional variable cost for electricity, without having to pay grid fees, given the small size of the boiler (assuming that the capacity was already booked and paid for).
The current grid fee structure with fixed fees of about €20,000 per megavolt-amperes (MVA) requires at least 3,000 hours of low-cost electricity to enable electrification at scale. However, in many cases, the transmission grid still has capacity, and market building, dynamic pricing, and local grid price-setting based on congestion can create a win–win–win (as the grid gets money for capacity not used, industrial players receive cheap heat, and the electricity market has a higher floor).
Companies can capture the electrification opportunity with an integrated approach
Companies can be proactive in overcoming perceived challenges to electrification by considering four actions.
- Develop a vision and a case for action: Companies with a committed leadership focus and vision for the energy transition could benefit from more cost-effective, sustainable, and resilient energy solutions, including cases with a demonstrated positive net present value (NPV). These solutions can not only improve short-term cost competitiveness and resilience toward energy and carbon price swings but can also fund the subsequent steps of the transition. First movers may gain an additional advantage through green premiums on their products.
- Build capabilities and constantly renew them: To accelerate progress at a lower cost, companies can establish a center of excellence that provides standardized engineering and a centralized platform for sharing best practices. This platform can be boosted by a dedicated technology search function to identify emerging solutions and opportunities for collaborative operating models. Approaches can be driven by a holistic mindset and theoretical limits, rather than incremental improvements. Key capabilities for the cross-functional team can include performance management, advanced analytics, process redesign, heat integration, and sourcing of green energy.
- Implement rigorous delivery models and governance: Companies require a rigorous cadence of project planning and implementation to deliver value, codify methodology, and roll out high-impact, repeatable initiatives. Companies can deliver project management approaches to progress and risks in an objective and consistent manner across sites. Bottom-up planning supported by structured, daily performance dialogues can drive team productivity.
- Be bold and use what exists: Dare to come with completely new designs by pushing the boundaries and opening the aperture. By using existing funds intended for technology development and decarbonization, industrials can realize bold visions.
Electrifying industrial heat is less challenging than previously believed. Although industrial heat requirements vary widely, commercially available technologies and bespoke design solutions are rapidly filling the gaps. As economic feasibility increases, companies that commit to decarbonization could benefit from both cost-efficiency and emissions reductions on the path to net zero.