What if a technological breakthrough could help the power sector decarbonize—and help prevent the worst effects of climate change?
Power generation currently accounts for approximately 30 percent of global CO2 emissions. To meet the Paris Agreement’s target of full decarbonization by 2050, many governments and utilities are shifting away from fossil fuels as a primary energy source and turning to renewable-energy technologies. The goal for many power sector players and their regulators is a zero-carbon energy grid. Volatility in the energy markets and geopolitical challenges may have complicated the transition to net zero in the short run, but in the longer run, the economics of renewable-power sources will drive likely investment into them.
In addition, as other industries transition away from fossil fuels, the demand for zero- or low-carbon electricity will increase. For example, as electric vehicles replace internal-combustion vehicles, more electricity generation will be required. McKinsey’s Global Energy Perspective 2022 projects that power consumption could triple by 2050. For countries to hit their decarbonization goals, it is thus essential that not just existing but also all added generation be zero carbon.
Renewable energy from wind and solar is currently the most cost-efficient form of new zero-carbon electrical generation, and by 2030 it is expected be the lowest-cost of any kind of generation in most markets. The continued development of wind and solar technologies and construction techniques is expected to continue, which means that the bulk of new near-term clean-electricity generation will probably come from these two sources. But wind and solar have their limitations: they are nondispatchable—that is, they generate electricity when the wind blows or the sun shines, not necessarily when the grid needs it. One example of such limitations: energy shortages in Europe that began in 2019—before Russia’s invasion of Ukraine—that were partly caused by historically low wind speeds lasting for months.
Other forms of dispatchable zero-carbon energy, such as geothermal or tidal power, are encouraging. But they are generally more expensive than wind and solar, can function only in a limited number of sites, and are less technologically mature. Grid-scale batteries and other forms of energy storage are increasingly promising, but they are still cost prohibitive at the required durations and have not yet reached the level of technological readiness for large-scale deployment.
Nuclear-fusion energy could help provide flexibility for zero-carbon electricity grids. Fusion—different from nuclear fission, which releases energy by splitting an atom in two—creates energy by combining two atoms, typically hydrogen isotopes. Fusion is dispatchable, which means that, unlike wind and solar, it does not rely on environmental or other external variables to generate power. The process of producing fusion energy creates no carbon emissions and no long-lived nuclear waste from spent fuel.1
Historically, fusion machines have not been technically viable, because the energy input required to power the reaction has been larger than the energy produced by the machine. But in the last five years, fusion energy has reached a turning point in its development. Our analysis suggests that it could potentially play a large role in meeting 2050 decarbonization targets and may be worth considering in policies, plans, and investments.
A zero-carbon energy grid requires flexibility
A grid that relies on nondispatchable energy sources, like wind and solar, typically requires alternative sources of power generation. In other words, the minute-by-minute matching of the supply of wind and solar power to demand cannot occur in the way that energy from baseload-generating plants fueled by coal or natural gas can. Wind and solar are therefore sometimes referred to as variable renewable energy (VRE). A zero-carbon electrical grid cannot operate in a stable way with 100 percent VRE and will require sources of flexibility to back up, or firm, the VRE generation. In addition, land use for VRE and the required transmission infrastructure to connect it are increasing concerns. In Germany, for example, the construction of wind projects has not only slowed but also failed to achieve deployment goals for the past four years.
Flexibility—the ability to manage the intermittency of nondispatchable wind and solar—is thus crucial to achieving a zero-carbon energy grid. The real-time matching of supply and demand can be ensured in a number of ways. Gas and coal plants can adjust production up or down to smooth out fluctuations in the output of wind and solar power, for example, but relying on fossil fuels is not acceptable in a zero-carbon generation system unless carbon capture technology is used. Transmission lines can balance production across geographies. Well-designed incentives can encourage users to modify their consumption via demand-side management programs. Battery storage can serve the power system as both a generator (when discharging) and a consumption point, or load (when charging). This need for flexibility is creating a wave of innovation across different technologies, including dozens of types of batteries and other energy storage technologies, natural-gas combustion with carbon sequestration, and digitally enabled demand-side aggregators.
To determine the optimal mix of zero-carbon technologies in a given region while preserving the reliability of the system, researchers and energy planners can use techno-economic grid modeling. Our research, using this technique, shows that the most valuable resources of a decarbonized grid are dispatchable zero-carbon generation sources. Unlike batteries or other electricity storage devices, which must be charged from the grid, these technologies generate net electrical energy and thus play a unique role in a decarbonized grid: they can be turned on during periods of low wind and solar output, without the duration constraints of batteries and with a high assurance of availability. Our modeling suggests that if this kind of dispatchable zero-carbon generation were available at a sufficiently low cost, the energy system in Europe, for example, could consist2 almost entirely of some VRE plus dispatchable sources (Exhibit 1). Finding an affordable, scalable, and safe dispatchable zero-carbon generation technology would constitute a gigantic step toward a sustainable energy future for humanity. Fusion is potentially one of these technologies.
Fusion energy is potentially at a turning point
Fusion energy, also known as controlled nuclear fusion, has been pursued since the 1950s, first as a classified program and then, since a landmark conference in 1958, as an open, collaborative international effort. Simply put, fusion works by combining light atoms, such as hydrogen, into heavier products, such as helium. The reaction releases enormous amounts of energy, which is then captured and converted into useful electricity by a fusion machine (Exhibit 2). There are many fusion machine designs, such as magnetic confinement (tokamaks and stellarators), inertial confinement, and magnetized target fusion.
Fusion energy has some critical advantages over fission as a zero-carbon power source. It is fully controllable and thus creates dispatchable power with a ramp rate fast enough to complement renewables in a VRE-heavy grid. The fuel is readily available to all nations—for example, the required hydrogen isotopes for one commonly proposed fusion reaction can be extracted from seawater3; some companies, such as TAE Technologies, are proposing fuel cycles using ordinary materials like hydrogen and boron.4 The fuel is slowly metered into the machine in a way that makes meltdowns or runaway events essentially impossible.5 And fusion creates no carbon emissions and minimal nuclear waste—only the vessel itself.6 Unlike today’s fission plants, fusion produces no long-lived fuel waste.
These advantages are so substantial that fusion energy has long seemed the holy grail of energy technology. However, creating the temperature and pressure conditions required to initiate the reaction in the fusion machine is a great scientific and technological challenge—so much so that after more than 60 years of experimentation, a marketable solution remains elusive. Skeptics point out that practical fusion energy has been 20 years away for the past 50 years. The basic problem is the difficulty of preventing energy from leaking out, which means that fusion machines, so far, consume more energy than they create.
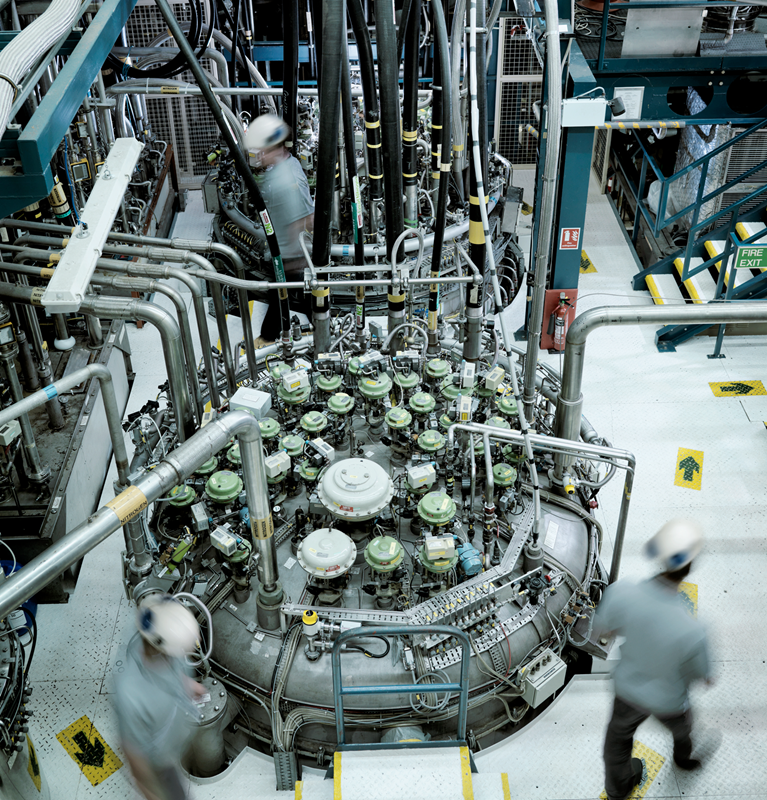
Creating the right temperature and pressure conditions in a fusion machine has been a scientific challenge for decades. Enabling technologies have allowed fusion to break new barriers.
In addition, the machines are complex: they require the world’s most advanced magnets, hard-to-engineer materials that can withstand the intense temperatures on the machine’s inside wall, and submillimeter precision for machined parts several meters across. Conceptual designs, when costed out, have seemed too expensive to be competitive with other forms of generation. From the 1970s onward, governmental enthusiasm for funding fusion projects has therefore waned.
But now, there are reasons to believe that fusion energy could be at a turning point. These include the following:
- The development of enabling technologies has allowed fusion to break new barriers. For example, additive manufacturing—often called 3-D printing—allows the complex geometrical shapes of parts required for the walls of fusion machines to be produced at low cost and designs to be iterated quickly. Rapidly increasing computer capacity has made it possible for simulation codes to represent fusion reactions in greater detail, so predictions about performance can be made without the expense of building large experiments. And rapid digital controls are improving the suppression of the vibrations that cause energy to leak out of the core fusion reaction. These and other technological advances have created the conditions in which fusion can develop more rapidly. In the same way, advancing lithium-ion battery technology has allowed electric vehicles to start proliferating after failing to achieve widespread adoption for 100 years, and manufacturing breakthroughs in low-cost polysilicon solar panels (by Chinese and other OEMs) from 2005 to 2008 largely enabled the massive scale-up of solar deployment in the past decade.
- There has been a sea change in the orientation of fusion research programs. Most fusion research used to occur in science-oriented, publicly funded labs. Now there is a new wave of privately backed programs, as well as programs working toward commercial viability in both the public and private sectors. This development has been driven partly by the fact that enabling technologies have progressed enough for private investors to step in with confidence. The result is that numerous privately funded start-ups now hope to operate commercial fusion machines before the end of the 2020s. A few major public labs are also launching programs to build commercially viable operational fusion machines. For example, the United Kingdom’s STEP program (out of the fusion research center at Culham) seeks to build a new fusion machine by 2040, and the Chinese fusion program recently accelerated plans for the China Fusion Engineering Testing Reactor, a prototype commercial fusion machine.
- Investment in private fusion funding has greatly accelerated. Private investment in fusion energy has surged over the past 20 years, with the value of investments nearly tripling in 2021 (Exhibit 3). This increased funding is a combination of traditional technology venture funding; strategic investments by incumbent energy companies, such as Eni’s and Equinor’s investments in Commonwealth Fusion Systems; seed investments by ultra-high-net-worth individuals, such as Sam Altman’s 2021 investment in Helion Energy; and government investments, such as the participation of the UK Innovation & Science Seed Fund in Tokamak Energy’s series A venture round. Access to capital is allowing private companies to construct larger components for fusion machines and to design full-scale prototypes for construction later this decade.
The next five to ten years will be critical for the development of fusion energy
Some key benchmarks over the next decade will help indicate whether fusion is truly on a trajectory toward implementation. Over the next five years, we would expect to see these advances:
- Demonstration of the core temperature and pressure conditions required for the fusion energy produced to exceed the heating energy injected into the reaction. The temperatures required to produce energy from a fusion reaction are on the order of 50 million degrees Celsius. The hotter the core of a fusion machine can get, and the more pressure it can withstand without leaking energy, the more net energy it can produce. This level of confinement, long a challenge in achieving net energy gain, is a necessity.
- Confirmation of component-level performance of various fusion concepts. By 2025, leading private fusion players plan to demonstrate, or in some cases have already demonstrated, major subsystems. These include powerful high-temperature superconducting magnets (achieved by Commonwealth Fusion Systems in 2021), plasma injectors (such as the P13 injector demonstrated by General Fusion in 2017), radio frequency heating systems, and new wall materials that can survive the intense heat of a fusion machine’s interior. Successful tests of these major subsystems and components by 2025 would mean that operational prototype plants could be functioning by the decade’s end.
- Demonstration of fusion in conditions relevant for power plants and validation of the economics. By 2026, we would expect to see at least one player integrating all major subsystems into a functioning prototype that can validate system level performance. Such a prototype would also make it possible, for the first time, to conduct a Class 4 (feasibility-study level) estimate of the costs of a fusion machine’s parts manufacturing and assembly. This would be the first model of a fusion power plant’s economics that could truly inspire confidence.
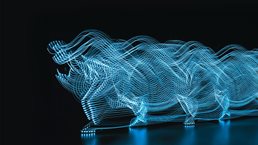
The new digital edge: Rethinking strategy for the postpandemic era
For fusion to play a major role in decarbonization before 2050, other key elements will also need to be in place within the next ten years:
- A regulatory framework for licensing fusion power plants. As we have said, fusion machines are inherently less susceptible to serious safety incidents than nuclear-fission reactors. But it is unclear whether fusion machines will be licensed under fission frameworks, which are designed for nuclear plants based on existing fission technology and involve years of intensive design reviews.7 The countries that first deploy fusion machines will need a regulatory framework designed to allow rapid development while ensuring safety. For example, in the autumn of 2021, the United Kingdom, which seeks to make fusion a part of its national decarbonization plans, embarked on an effort to develop a made-for-fusion licensing framework.8 It will take into account the technical characteristics of fusion devices and allow the rapid deployment of fusion once a machine’s design has been proved. For comparison, countries providing for flexibility in the traditionally heavily regulated rocket and spacecraft sector are seeing innovations from the private sector.
- The beginning of a scaled global supply chain and workforce for fusion energy. From lithium fuel–producing modules to advanced radio frequency heating sources, fusion will require specialized parts now fabricated one-off for laboratory experiments. Increasing confidence in the viability of fusion could stimulate investments in supply chains that support the construction of multiple fusion power plants. In addition, engineers, construction workers, and plant operators will have to be trained through academic programs and apprenticeships.
- The first commercial power plants with traditional project finance. Projects funded by equity investors, along with construction loans from banks and other financiers, would be a strong indication that fusion is on a path to adoption. There may be some de-risking loans by governments in the early days, but project development will not need to rely on the massive governmental de-risking loans and grants that fission plants have lately required in the West.
For leaders in business, financial management, and government, this is the time to start preparing for the development of fusion energy. Leaders can start to plan for the opportunities and risks by considering several moves:
- Understand how the development of a working fusion machine could disrupt energy investments. Techno-economic modeling can indicate the conditions under which fusion could supplant or complement battery storage, gas power using carbon capture, and other potentially less cost-effective technologies. Including fusion in at least some investment scenarios can give leaders a fuller picture of potential decarbonization pathways.
- Consider the degree to which fusion should be built into decarbonization plans. Fusion energy is not a sure thing—there are still major technical challenges, such as the performance of the magnets, the survivability of the first wall, plasma stability in confinement concepts other than tokamaks, and reliable heating systems. However, the potential upside could be great enough to make evaluating whether fusion should have a place in corporate or national energy plans worthwhile. A fusion-heavy grid would imply major shifts in investment over the next 30 years, including a partial shift from wind and solar with battery storage toward fusion machines. Such a grid will also require policy changes for the licensing of facilities. It is therefore worth starting the conversation today—these changes will take years to implement.
- Understand the value chain of fusion energy. Fusion power plants, like any complex system, will require a supply chain of parts and a value chain to develop and operate. That includes early-stage development, final development, construction, financing, operation, and end-of-life decommissioning. Leaders should begin to understand these supply and value chains to identify the potential control points and investment opportunities. Such preparations will lay the groundwork for capturing fusion’s financial and strategic upside as the technology is proved and the industry ramps up in size. Of course, it will be necessary to recognize that the technology must advance further before an industry can grow up around it.
The technology still needs to develop, and there is no guarantee that recent fusion concepts will ultimately produce net energy. Yet this time may be different for the longtime dream of fusion energy. Fusion’s characteristics as a zero-carbon, dispatchable source of electricity would allow it to play a key role in grids, along with wind, solar, carbon capture, and other technologies. For all leaders—especially policy makers and leaders in the energy sector and industries that are large consumers of energy—being prepared is prudent.