In the Paris Agreement of 2015, member states agreed to limit global warming to 2 °C versus pre-industrial levels. This would imply reducing greenhouse gas (GHG) emissions by 80 to 95 percent of the 1990 level by 2050. As industry accounted for about 28 percent of global greenhouse gas emissions in 2014, it follows that these targets cannot be reached without decarbonizing industrial activities. Industrial sites have long lifetimes; therefore, upgrading or replacing these facilities to lower carbon emissions requires that planning and investments start well in advance.
Stay current on your favorite topics
In this report, we investigate options to decarbonize industrial processes, especially in the cement, steel, ethylene, and ammonia sectors. We selected these sectors because they are hard to abate, due to their relatively high share of emissions from feedstocks and high-temperature heat compared to other sectors. We conclude that decarbonizing industry is technically possible, even though technical and economical hurdles arise. We also identify the drivers of costs associated with decarbonization and the impact it will have on the broader energy system.
The industrial sector is both a global economic powerhouse and a major emitter of GHG emissions
The industrial sector is a vital source of wealth, prosperity, and social value on a global scale. Industrial companies produce about one-quarter of global GDP and employment, and make materials and goods that are integral to our daily lives, such as fertilizer to feed the growing global population, steel and plastics for the cars we drive, and cement for the buildings we live and work in.
In 2014, direct GHG emissions from industrial processes and indirect GHG emissions from generating the electricity used in industry made up ~15 Gton CO2e (~28 percent) of global GHG emissions. CO2 comprises over 90 percent of direct and indirect GHG emissions from industrial processes. Between 1990 and 2014, GHG emissions from the industrial sector increased by 69 percent (2.2 percent per year)1, while emissions from other sectors such as power, transport, and buildings increased by 23 percent (0.9 percent per year).2
Almost 45 percent of industry’s CO2 emissions result from the manufacturing of cement (3 Gton CO2), steel (2.9 Gton CO2), ammonia (0.5 Gton CO2), and ethylene (0.2 Gton CO2)—the four sectors that are the focus of this report. In these four production processes, about 45 percent of CO2 emissions come from feedstocks, which are the raw materials that companies process into industrial products (for example, limestone in cement production and natural gas in ammonia production). Another 35 percent of CO2 emissions come from burning fuel to generate high-temperature heat. The remaining 20 percent of CO2 emissions are the result of other energy requirements: either the onsite burning of fossil fuels to produce medium- or low-temperature heat, and other uses on the industrial site (about 13 percent) or machine drive (about 7 percent) (see Exhibit 1).3
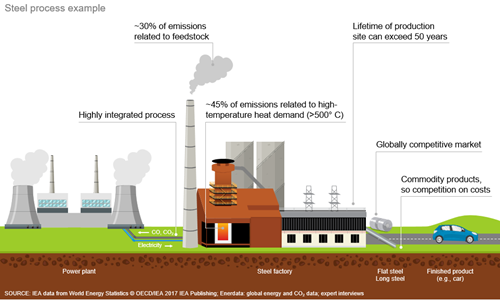
After breakthroughs in the power, transport, and buildings sectors, industrial decarbonization is the next frontier
Global efforts have driven innovation and the scaling up of decarbonization technologies for the power, buildings, and transport sectors. This has led to major reductions in the costs of these technologies. Examples are the recent reductions in the costs of solar photovoltaic modules and electric vehicles. Less innovation and cost reduction have taken place for industrial decarbonization technologies. This makes the pathways for reducing industrial CO2 emissions less clear than they are for other sectors.
Besides that, CO2 emissions in the four focus sectors are hard to abate for four technical reasons. First, the 45 percent of CO2 emissions that result from feedstocks cannot be abated by a change in fuels, only by changes to processes. Second, 35 percent of emissions come from burning fossil fuels to generate high-temperature heat (in the focus sectors, process temperatures can reach 700 °C to over 1,600 °C). Abating these emissions by switching to alternative fuels such as zero-carbon electricity would be difficult, because this would require significant changes to the furnace design. Third, industrial processes are highly integrated, so any change to one part of a process must be accompanied by changes to other parts of that process. Finally, production facilities have long lifetimes, typically exceeding 50 years (with regular maintenance). Changing processes at existing sites requires costly rebuilds or retrofits.
Economic factors add to the challenge. Cement, steel, ammonia, and ethylene are commodity products for which cost is the decisive consideration in purchasing decisions. With the exception of cement, these products are traded globally. Generally, across all four sectors, externalities are not priced in and the willingness to pay more for a sustainable or decarbonized product is not yet there. Therefore, companies that increase their production costs by adopting low-carbon processes and technologies will find themselves at an economic disadvantage to industrial producers that do not.
Industrial companies can reduce CO2 emissions in various ways, with the optimum local mix depending on the availability of biomass, carbon-storage capacity and low-cost zero-carbon electricity and hydrogen, as well as projection changes in production capacity
A combination of decarbonization technologies could bring industry emissions close to zero: demand-side measures, energy efficiency improvements, electrification of heat, using hydrogen (made with zero-carbon electricity) as feedstock or fuel, using biomass as feedstock or fuel, carbon capture and storage (CCS), and other innovations.4
The optimum mix of decarbonization options depends greatly on local factors. The most important factors are access to low-cost zero-carbon electricity and access to a suitable kind of sustainably produced biomass, because most processes in the focus sectors have significant energy- and energy-carrier-related feedstock requirements that could be replaced by one or both of these alternatives. The local availability of carbon storage capacity and public and regulatory support for carbon storage determine whether CCS is an option. The regional growth outlook for the four focus sectors matters, too, because certain decarbonization options are cost effective for use at existing (brownfield) industrial facilities while others are more economical for newly built (greenfield) facilities.
Since the optimum combination of decarbonization options will vary greatly from one facility to the next, companies will need to evaluate their options on a site-specific basis. To help industrial companies narrow down their options and focus on the most promising ones, we offer the following observations, which account for current commodity prices and technologies (see Exhibit 2):
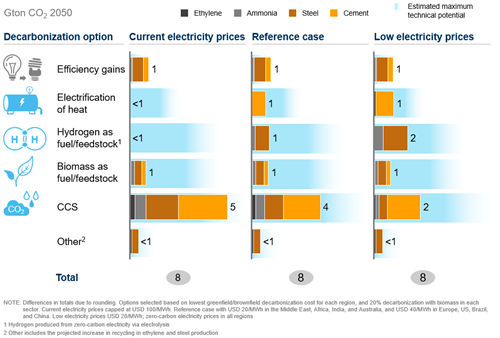
- Energy efficiency improvements can reduce carbon emissions competitively, but cannot lead to deep decarbonization on their own. Energy efficiency improvements that lower fuel consumption by 15 to 20 percent can be economical in the long run. However, depending on the payback times on energy efficiency required by companies (sometimes less than two years), implementation can be less than the potential of 15 to 20 percent.
- Where carbon-storage sites are available, CCS is the lowest-cost decarbonization option at current commodity prices. However, CCS is not necessarily a straightforward option for decarbonization. CCS imposes an additional operational cost on industrial companies, whereas further innovation could make alternative decarbonization options (for example, electrification of heat) cost competitive vis-à-vis conventional production technology. CCS can only be implemented in regions with adequate carbon-storage locations, and supportive local regulations and public opinion. CCS has the distinction of being the only technology that can currently fully abate process-related CO2 emissions from cement production.5
- At zero-carbon electricity prices below ~$50/MWh, using zero-carbon electricity6 for heat or using hydrogen based on zero-carbon electricity becomes more economical than CCS. Electricity prices below $50/MWh have already been achieved locally (eg, hydro and nuclear based power-system of Sweden) and could be achieved in more places with the current downward cost trend in renewable electricity generation. The minimum price that makes it less expensive to switch to zero-carbon electricity than to apply CCS for decarbonization depends strongly on the sector, local fossil fuel and other commodity prices and the state of the production site.
- At electricity prices below ~$50/MWh, electrifying heat production at greenfield cement plants is more cost-competitive than applying CCS to the emissions from fuel consumption, provided that very-high-temperature electric furnaces are available.78
- At electricity prices below ~$35/MWh, hydrogen use for greenfield ammonia and steel production sites is more cost-competitive than applying CCS to conventional production processes.
- At electricity prices below ~$25/MWh, electrification of heat in greenfield ethylene production and in brownfield cement production and usage of hydrogen for brownfield steel production are more cost-competitive than applying CCS to conventional production processes.
- Finally, below an electricity price of ~$15/MWh, usage of hydrogen for brownfield ammonia production and electrification of heat for ethylene production are more cost-competitive than applying CCS to conventional production processes. This means that electric heat production and usage of electricity to make hydrogen are more economical approaches to decarbonization than CCS in all four focus sectors at this electricity price level.
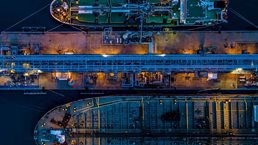
Powered by Energy Insights
Lower costs for capital equipment or process innovations could make electrification or the use of zero-carbon electricity based hydrogen economical at higher electricity prices.
- Using biomass as a fuel or feedstock is financially more attractive than the electrification of heat or the use of hydrogen in cement production and at electricity prices above ~$20/MWh in steel production. Mature technologies are available for using biomass as fuel and feedstock in steel and as fuel in cement production. These technologies reduce emissions more economically than CCS on the conventional process. Biomass can also replace fossil fuel feedstocks for ethylene and ammonia production. Though this approach costs more than electrification or hydrogen usage, it also abates emissions in both the process and at end-of-life of the product, such as the emissions from incineration of plastics made from ethylene. The global supply of sustainably produced biomass, however, is deemed limited at the global level. Additionally, re-forestation to generate offsets might be a counter use of biomass rather than the shipping and usage in industrial processes.
- Demand-side measures are effective for decarbonization but were not a focus of this report. Replacing conventional industrial products with lower-emission alternatives (eg, replacement of cement with wood for construction) would result in significant reductions in CO2emissions from the four focus sectors. Radical changes in consumption patterns driven by technology changes could further offset demand, such as reduced build-out of roads (and therefore cement) through autonomous driving, reduced demand for ammonia through precision agriculture. Moreover increasing the circularity of products, by eg, recycling or reusing them can also cut CO2emissions. Producing material based on recycled products generally consumes less energy and feedstock than production of virgin materials. As an example, producing steel from steel scrap requires only about a quarter of the energy required to produce virgin steel.
Industrial decarbonization will require increased investment in industrial sites and has to go hand in hand with an accelerated build-out of zero-carbon electricity generation
Completely decarbonizing the energy-intensive industrial processes in the four focus sectors will have a major impact on the energy system. It is estimated that it would require ~25 EJ to 55 EJ per year of low-cost zero-carbon electricity. In a business-as-usual world, only 6 EJ per year would be needed, indicating that, regardless of the mix of decarbonization options chosen, electricity consumption will go up significantly. The transition in the power and industrial sectors should thus go hand in hand. The industrial sector might be able to lower the costs of the power sector transition, eg, by providing grid balancing, while being a large off-taker that can support increased build-out of generation capacity.
The total costs of fully decarbonizing these four sectors globally are estimated to be ~$21 trillion between today and 2050. This can be lowered to ~$11 trillion if zero-carbon electricity prices come down further compared to fossil fuel prices (see Exhibit 3).9 These estimates are based on cost assumptions that do not allow for process innovations or significant reductions in the costs of capital equipment. Furthermore, they heavily depend on the emission reduction target, local commodity prices, the selected mix of decarbonization options, and the current state of the production site. The estimated costs for complete decarbonization of the four focus sectors are equivalent to a yearly cost of ~0.4 to 0.8 percent of global GDP ($78 trillion). According to the estimations in this report, about 50 to 60 percent of these costs consists of operating expenses and the remainder consists of capital expenditures, mainly for cement decarbonization.
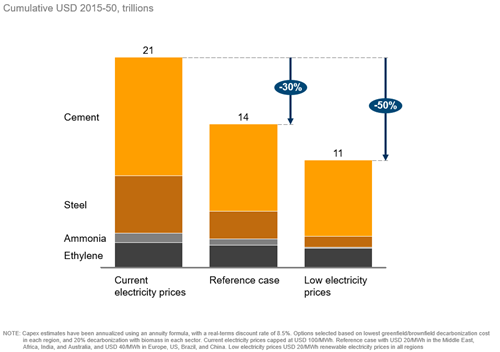
An analysis of the effects of different electricity prices suggests that decarbonization would have an upward impact on the costs of the industrial products: cement doubling in price, ethylene seeing a price increase of ~40 to 50 percent, and steel and ammonia experiencing a ~5 to 35 percent increase in price.10
Advance planning and timely action could drive technological maturation, lower the cost of industrial decarbonization and ensure the industry energy transition advances in parallel with required changes in energy supply
- Governments can develop roadmaps for industrial decarbonization on local and regional levels. Setting such a longer-term direction for decarbonization could support planning for decarbonization by other parties, including industrial companies, utilities and owners of key infrastructure (such as the electricity grid or hydrogen pipelines), and unlock investments with long payback times. Such a roadmap should take a perspective, eg, on the production outlook, resource availability (including carbon-storage sites), additional resources required (zero-carbon electricity generation, etc.), coordinated roll-out of infrastructure and demand-side measures, as well as the role government would play (eg, in the development of critical infrastructure).
- Adjust regulation and incentives in line with decarbonization roadmaps. Various policy mechanisms could support industrial decarbonization. These might include direct incentives for companies to decarbonize, or adjustments to the financial requirements placed on utilities and other companies involved in energy generation and distribution.
- Industrial companies should prepare for decarbonization by conducting a detailed review of each facility in their portfolio. Such a review should include the availability of low-cost zero-carbon electricity, zero-carbon hydrogen, biomass, and carbon-storage capacity near the facility as these will differ on a country-by-country basis. Interaction with other stakeholders, such as governments, utilities, and other industrial companies, could help to identify synergies between industrial decarbonization and decarbonization in other sectors or companies, driving targeted innovation and driving down costs. For example, companies in an industrial cluster might benefit from shared carbon-storage infrastructure.
- Governments, industrial companies, and research institutions can support innovation and the scale up of promising decarbonization technologies, which is required to reach full decarbonization of the industrial sector. Innovative decarbonization technologies could potentially lower the costs of the industry transition. Governments can support the development of innovative decarbonization options, including the scale up of global markets, eg, in certain types of biomass, or the introduction of innovative processes to lower implementation costs. Overall, decarbonizing industrial sectors requires collaboration across governments, industrial players, and research institutes, similar to the effort that led to the cost reduction and scale up of renewable energy generation.