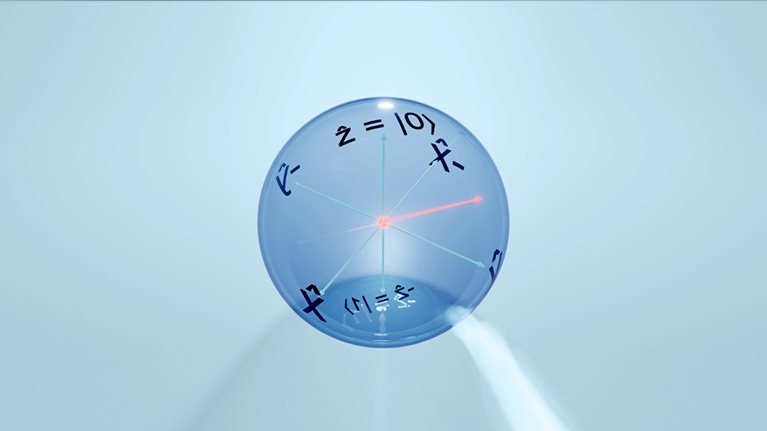
Flip a coin. Heads or tails, right? Sure, once we see how the coin lands. But while the coin is still spinning in the air, it’s neither heads nor tails. It’s some probability of both.
This gray area is the simplified foundation of quantum computing.
Get to know and directly engage with senior McKinsey experts on quantum computing
Ondrej Burkacky is a senior partner in McKinsey’s Munich office, Miklós Gábor Dietz is a senior partner in the Vancouver office, Dieter Kiewell and Jared Moon are senior partners in the London office, Alexandre Ménard is a senior partner in the Paris office, Mark Patel is a senior partner in the Bay Area office, and Rodney Zemmel is a senior partner in the New York office.
Digital computers have been making it easier for us to process information for decades. But quantum computers are poised to take computing to a whole new level. Quantum computers represent a completely new approach to computing. They have the potential to solve very complex statistical problems that are beyond the limits of today’s computers. Quantum computing has so much promise and momentum that McKinsey has identified it as one of the next big trends in tech. Quantum computing alone—just one of three main areas of emerging quantum technology—could account for nearly $1.3 trillion in value by 2035. Investors of all kinds are perking up their ears—and opening up their wallets: government investors alone have pledged $34 billion in investments. In 2022, the US government announced $1.8 billion in funding, bringing its total investment to $3.7 billion.
Learn more about McKinsey Digital.
How does a quantum computer work?
Here’s how quantum computing works: classical computing, the technology that powers your laptop and smartphone, is built on bits. A bit is a unit of information that can store either a zero or a one. By contrast, quantum computing is built on quantum bits, or qubits, which can store zeros and ones. Qubits can represent any combination of both zero and one simultaneously—this is called superposition, and it is a basic feature of any quantum state. Chips are the physical hardware that store qubits, just like in classical computing.
When a classical computer solves a problem with multiple variables, it must conduct a new calculation every time a variable changes. Each calculation is a single path to a single result. Quantum computers, however, can explore many paths in parallel through superposition.
Additionally, qubits can interact with one another. This is known as entanglement. Entanglement allows qubits to scale exponentially; two qubits, for example, can store and process four bits of information, three can process eight, and so on. This exponential scaling gives the quantum computer much more power than classical computers.
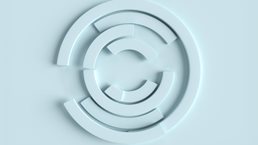
Looking for direct answers to other complex questions?
Heavyweight tech organizations are already placing bets on quantum technology. In 2019, Google claimed that its quantum computer had solved in just 200 seconds a problem that would have taken a classical computer 10,000 years (although other tech organizations and academics have surfaced doubts about the validity of Google’s claim).
Even if Google’s claim was accurate, the achievement was more of a theoretical leap forward than a practical one since the problem its quantum computer solved had no real-world use. But we’re rapidly approaching a time when quantum computers will have a real impact on our lives.
What are quantum computers used for?
Today’s classical computers are relatively straightforward. They work with a limited set of inputs and use an algorithm and spit out an answer—and the bits that encode the inputs do not share information about one another. Quantum computers are different. For one thing, when data are input into the qubits, the qubits interact with other qubits, allowing for many different calculations to be done simultaneously. This is why quantum computers are able to work so much faster than classical computers. But that’s not the end of the story: quantum computers don’t deliver just one clear answer like classical computers do; rather, they deliver a range of possible answers.
For calculations that are limited in scope, classical computers are still the preferred tools. But for very complex problems, quantum computers can save time by narrowing down the range of possible answers.
When will quantum computers be available?
Over the next few years, the major players in quantum computing, as well as a small cohort of start-ups, will steadily increase the number of qubits that their computers can handle and improve how the technology functions. Progress in quantum computing, however, is expected to remain slow. According to our conversations with tech executives, investors, and academics in quantum computing, 72 percent believe we’ll see a fully fault-tolerant quantum computer by 2035. The remaining 28 percent think this milestone won’t be reached until 2040 or later.
But some businesses will begin to derive value from quantum well before then. At first, businesses will receive quantum services via the cloud. Several major computing companies have already announced their quantum cloud offerings.
Learn more about McKinsey Digital.
What are some obstacles that impede the development of quantum computing?
One major obstacle to the advancement of quantum computing is that qubits are volatile. Whereas a bit in today’s classical computers is in a state of either one or zero, a qubit can be any possible combination of the two. When a qubit changes its status, inputs can be lost or altered, throwing off the accuracy of the results. Another obstacle to development is that a quantum computer operating at the scale needed to deliver significant breakthroughs will require potentially millions of qubits to be connected. The few quantum computers that exist today are nowhere near that number.
Here are some other challenges facing the technologies that could support quantum computing at scale:
- High-fidelity two-qubit gates at scale. Maintaining high fidelity (meaning accuracy and reliability greater than 99.99 percent) is required for fault-tolerant quantum computers. Doing so at scale will be difficult.
- Speed. Qubits need to retain their quantum state to be able to interact with one another. Even in specific environmental conditions, they will eventually degrade. For quantum computers to operate at scale, gate operations will need to move very quickly to complete computations before qubits degrade.
- Multiqubit networking. Connecting, or networking, qubits to one another could theoretically make quantum computers much more powerful. The key challenge here is connecting qubits across chips, or from one physical quantum computer to another.
- Individual qubit control at scale. The control of individual qubits becomes increasingly complex as the number of qubits increases.
- Cooling power and environmental control. As quantum computers become larger, the size and power requirements of the cooling equipment become more and more expensive, from both a cost and an environmental standpoint. Currently, powering a quantum computer large enough to connect millions of qubits is cost prohibitive.
- Manufacturability. Producing large numbers of quantum computers will require automating both the manufacturing and testing processes. The production of certain quantum computers may require developing entirely new manufacturing techniques.
How can classical computers and quantum computers work together?
Slowly, at first. Initially, quantum computing will be used alongside classical computing to solve multivariable problems. One example? Quantum computers can narrow the range of possible solutions to a finance or logistics problem, helping a company reach the best solution faster. This kind of slower progress will be the norm until quantum computing advances enough to deliver more significant breakthroughs.
Learn more about McKinsey Digital.
What are some potential business use cases for quantum computers?
Quantum computers have four fundamental capabilities that differentiate them from today’s classical computers:
- Quantum simulation. Quantum computers can model complex molecules, which may eventually help reduce development time for chemical and pharmaceutical companies. Scientists looking to develop new drugs need to examine the structure of a molecule to understand how it will interact with other molecules. It’s almost impossible for today’s computers to provide accurate simulations, because each atom interacts with other atoms in complex ways. But experts believe that quantum computers are powerful enough to eventually be able to model even the most complex molecules in the human body. This opens up the possibility for faster development of new drugs and new, transformative cures.
- Optimization and search. Every industry relies in one way or another on optimization. Where are robots best placed on a factory floor? What’s the shortest route for a company’s delivery trucks? There are almost infinite questions that need to be answered to optimize for efficiency and value creation. With classical computing, companies must make one complicated calculation after another, which can be a time-consuming and costly process given the many potential variables of a situation. Since a quantum computer is able to work with multiple variables simultaneously, it can be used to quickly narrow the range of possible answers. From there, classical computing can be used to zero in on one precise answer.
- Quantum AI. Quantum computers have the potential to work with better algorithms that could transform machine learning across a diverse range of industries, from automotive to pharmaceuticals. In particular, quantum computers could accelerate the arrival of self-driving vehicles. Companies like Ford, GM, Volkswagen, and numerous mobility start-ups are running video and image data through complex neural networks. Their goal? To use AI to teach a car to make crucial driving decisions. Quantum computers’ ability to perform multiple complex calculations with many variables simultaneously allows for faster training of such AI systems.
- Prime factorization. Businesses today use large, complex prime numbers as the basis for their encryption efforts, numbers too large for classical computers to process. Quantum computing will be able to use algorithms to solve these complex prime numbers easily, a process called prime factorization. (In fact, a quantum algorithm known as Shor’s algorithm theoretically already can; there’s just not a computer powerful enough to run it.) Once quantum computers have advanced enough, new quantum-encryption technologies will be needed to protect online services. Scientists are already at work on quantum cryptography to prepare for this eventuality. McKinsey estimates quantum computers will be powerful enough for prime factorization by the late 2020s at the very earliest.
As these capabilities develop at pace with quantum computing power, use cases will proliferate.
What industries stand to benefit the most from quantum computing?
Research suggests that several industries in particular stand to reap the greatest short-term benefits from quantum computing based on the use cases discussed in the previous section. Collectively—and conservatively—the value at stake for these industries could be in the trillions of dollars.
- Pharmaceuticals. Quantum computing has the potential to revolutionize the research and development of molecular structures in the biopharmaceuticals industry. With quantum technologies, research and development for drugs could become less reliant on trial and error, and therefore more efficient. (Read more on how quantum computing stands to affect the pharmaceutical industry.)
- Chemicals. Quantum computing could be used to improve catalyst design, which could enable savings on existing production processes. Innovative catalysts could also enable the replacement of petrochemicals with more sustainable feedstock or the breakdown of carbon for CO2 usage. (Read more on how quantum computing might affect the chemicals industry.)
- Mobility. Quantum computing could result in a mobility ecosystem that is fully connected, intelligent, and environmentally friendly. Changes depend on the rapid and smooth exchange of vast amounts of data between in-vehicle computers and computers elsewhere. Quantum computers can process these large amounts of data in ways existing computers can’t, making this type of data exchange a realistic possibility. (Read more about how quantum technologies could affect the mobility industry.)
- Automotive. Within the mobility sector, the automotive industry could benefit from quantum computing in its R&D, product design, supply chain management, production, and mobility and traffic management. For example, quantum computing could be applied to decrease manufacturing costs by optimizing complex multirobot processes including welding, gluing, and painting. (Read more about how quantum technologies could affect the automotive industry.)
- Finance. Quantum computing use cases in finance are slightly further in the future. The long-term promise of quantum computing in finance lies in portfolio and risk management. One example could be quantum-optimized loan portfolios that focus on collateral to allow lenders to improve their offerings. By the time a fault-tolerant quantum computer is available, we estimate that the use cases in finance could create $622 billion in value. (Read more about how quantum computing could affect financial services.)
These five industries likely stand to gain the most from quantum computing, at least at first. But leaders in every sector can—and should—prepare for the inevitable quantum advancements of the coming years.
Learn more about McKinsey Digital.
What are the other quantum technologies aside from computing?
According to McKinsey’s analysis, quantum computing is still years away from widespread commercial application. Other quantum technologies such as quantum communication (QComms) and quantum sensing (QS) could become available much earlier.
Quantum communication will enable strong encryption protocols that could greatly increase the security of sensitive information. QComms can help facilitate the following functions:
- Full security when information is transferred between locations. Quantum-encryption protocols are more secure than classical protocols, most of which will likely be able to be broken once quantum computers attain more computing power or can work with more efficient algorithms.
- Enhanced quantum computing power in two important types of quantum processing: parallel quantum processing (where multiple processors are connected and simultaneously execute different calculations from the same problem) and blind quantum computing (where quantum communications provide access to remote, large-scale quantum computers in the cloud). Both types of processing are made possible by the entanglement of quantum particles. Entanglement is when quantum particles like qubits have connected properties, which means one particle’s properties can be manipulated by actions done to another.
Quantum sensing allows for more accurate measurements than ever before, including of physical properties like temperature, magnetic fields, and rotation. Plus, once optimized and decreased in size, quantum sensors will be able to measure data that can’t be captured by current sensors.
The markets for QComms and QS are currently smaller than the market for quantum computing, which has so far attracted most of the headlines and funding. But McKinsey expects both Qcomms and QS to attract serious interest and funding in the future. The risks are significant, but the potential payoff is high: by 2030, QS and QComms could generate $13 billion in revenues.
Learn more about quantum sensors and quantum communications.
How can organizations ensure that they have the quantum computing talent they need?
A wide talent gap exists between the business need for quantum computing and the number of quantum professionals available to meet that need. This skills gap could jeopardize potential value creation, which McKinsey estimates to be as much as $1.3 trillion.
The talent gap is felt differently by companies of different sizes. Small start-ups working in the quantum space typically grow out of university research labs and often have direct access to skilled candidates. Larger companies might have less of a connection to these talent pools.
McKinsey research has found that there is only one qualified quantum candidate for every three quantum job openings. By 2025, McKinsey predicts that less than 50 percent of quantum jobs will be filled unless there are significant changes to the talent pool or predicted rate of quantum-job creation.
Here are five lessons derived from the AI talent journey that can help organizations build the quantum talent they need to capture value:
- Define your talent needs clearly. In the early days of AI, some organizations hired data scientists without a clear understanding of what skills were needed. To avoid making the same error with quantum, organizations should first identify possible fields of applications that a quantum computing team would work on and then ensure that new hires come from diverse backgrounds (reflecting best practices).
- Invest early in translators. As buzz built up around AI, the role of analytics translators became crucial to helping leaders identify and prioritize challenges best suited for AI to solve. With quantum, there’s a similar need: for translators with engineering, application, and scientific backgrounds who can help organizations understand the opportunities and players in the rapidly expanding ecosystem.
- Create pathways for a diverse talent pipeline. Many of the first AI models reflected the same biases that were present in the information that was used to train them. There often was also a lack of people with diverse perspectives and experience building and testing the models, which contributed to the bias issue. While it’s too early to know all the risks that could emerge from quantum, we can expect similar challenges if a diverse and empowered quantum workforce is not in place to work with this new technology. Efforts are needed at the university level, as well as in K–12 education.
- Build technology literacy for all. For employees at all levels of an organization to understand the potential of a new technology, they need a basic understanding of how it works and what it can do. With quantum, business leaders as well as workers up and down the supply chain, in marketing, IT infrastructure, finance, and more will require basic fluency in quantum topics.
- Don’t forget talent development strategies. Companies focus heavily on talent attraction during times of technological foment, but that’s just one piece of the talent puzzle. To retain specialists, companies need to carve out clear paths for talent development. One pharmaceutical company leans into both the purpose of its work—developing use cases that will help save lives—and the freedom it offers its team.
Learn more about McKinsey Digital and check out quantum computing job opportunities if you’re interested in working at McKinsey.
Articles referenced:
- “Quantum technology use cases as fuel for value in finance,” October 23, 2023, Martina Gschwendtner, Nicole Morgan, Henning Soller
- “Gearing up for mobility’s future with quantum computing,” September 13, 2023, Scarlett Gao, Timo Möller, Niko Mohr, Alexia Pastré, Felix Ziegler
- “Is winter coming? Quantum computing’s trajectory in the years ahead,” May 19, 2023, Scarlett Gao, Martina Gschwendtner, Hussein Hijazi, Nicole Morgan, Henning Soller
- “Quantum technology sees record investments, progress on talent gap,” April 24, 2023, Michael Bogobowicz, Scarlett Gao, Mateusz Masiowski, Niko Mohr, Henning Soller, Rodney Zemmel, Matija Zesko
- “Five lessons from AI on closing quantum’s talent gap—before it’s too late,” December 1, 2022, Niko Mohr, Kiera Peltz, Rodney Zemmel, and Matija Zesko
- “From basic research to market: Why the recent Nobel Prize in physics matters,” October 18, 2022, Mena Issler, Niko Mohr, and Henning Soller
- “Quantum computing funding remains strong, but talent gap raises concern,” June 15, 2022, Mateusz Masiowski, Niko Mohr, Henning Soller, and Matija Zesko
- “How quantum computing can help tackle global warming,” May 27, 2022, Jeremy O’Brien
- “Quantum computing just might save the planet,” May 19, 2022, Peter Cooper, Philipp Ernst, Dieter Kiewell, and Dickon Pinner
- “Shaping the long race in quantum communication and quantum sensing,” December 21, 2021, Gaurav Batra, Martina Gschwendtner, Ivan Ostojic, Andrea Queirolo, Hennig Soller, and Linde Wester
- “A quantum wake-up call for European CEOs,” December 20, 2021, Anika Pfalnzer, Wolf Richter, and Henning Soller
- “Quantum computing use cases are getting real—what you need to know,” December 14, 2021, Matteo Biondi, Anna Heid, Nicolaus Henke, Niko Mohr, Lorenzo Pautasso, Ivan Ostojic, Linde Wester, and Rodney Zemmel
- “Separating the wheat from the chaff: Quantum technology in an era of hype,” July 1, 2021, Victor Galitski, Dmitry Green, Benjamin Lev, Yuval Oreg, and Henning Soller
- “Pharma’s digital Rx: Quantum computing in drug research and development,” June 18, 2021, Matthias Evers, Anna Heid, and Ivan Ostojic
- “The current state of quantum computing: Between hype and revolution,” February 19, 2021, Lorenzo Pautasso, Anika Pflanzer, and Henning Soller
- “How quantum computing could change financial services,” December 18, 2020, Miklós Dietz, Nico Henke, Jared Moon, Jens Backes, Lorenzo Pautasso, and Zaheen Sadeque
- “Will quantum computing drive the automotive future?,” September 2, 2020, Ondrej Burkacky, Niko Mohr, and Lorenzo Pautasso
- “A game plan for quantum computing,” McKinsey Quarterly, February 6, 2020, Alexandre Ménard, Ivan Ostojic, Mark Patel, and Daniel Volz
- “The next big thing? Quantum computing’s potential impact on chemicals,” July 12, 2019, Florian Budde and Daniel Volz
This article was updated in April 2024; it was originally published in May 2023.
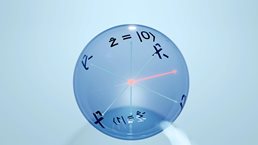