Based on the numbers alone, this appears to be the ideal time to be a semiconductor company. With chip demand soaring, annual revenue increased by 9 percent in 2020 and by 23 percent in 2021—far above the 5 percent reported in 2019. Even before the pandemic, capital markets were rewarding the industry’s surging profitability, with semiconductor companies delivering an annual average of 25 percent in total shareholder returns (TSR) from the end of 2015 to the end of 2019. Last year, shareholders saw even higher returns, averaging 50 percent per annum as remote work became the norm and consumers and businesses upped their technology purchases, helping to accelerate the digital revolution.
Behind the scenes, however, today’s semiconductor companies are facing a host of challenges. Even with fabs operating at full capacity, they have not been able to meet demand, resulting in product lead times of six months or longer. The ongoing semiconductor shortage now routinely makes headlines, especially when it forces automotive OEMs to delay vehicle production. What’s more, semiconductor companies are grappling with increased design complexity, a talent shortage, and pandemic-related issues that are disrupting the complex, global supply chain that links players in different markets. The shortage is now so concerning that it is prompting more large technology companies and major automotive OEMs to move chip design in-house—a trend that could have major implications for the market.
In other industries, manufacturers often respond to shortages by increasing output. But fab construction and production ramp-up for semiconductors are extremely costly and time consuming—often requiring a year for significant expansion or more than three years to build a new facility—which makes it difficult to increase semiconductor volumes quickly. While increasing capacity may sometimes help, it will not produce immediate results and typically requires a significant investment for many years before additional revenues—if any—will appear.
To help semiconductor companies develop a comprehensive plan for success, we quantified the benefits of capacity expansion to determine when construction may be justified. We also identified other strategies that can help semiconductor companies improve productivity and revenues, such as increasing the focus on leading-edge chips, pursuing innovations that go beyond node size, making bold long-term investments, developing greater resilience, improving the talent pipeline, and collaborating within the semiconductor ecosystem.
Understanding the semiconductor industry
Although headlines about the semiconductor shortage often discuss the situation in broad terms, the industry includes many different segments: memory, logic, analog, discrete, optical components, and sensors. Individual semiconductor companies and their manufacturing sites tend to focus on select segments. Their end customers, by contrast, typically require products from all semiconductor segments and thus rely on multiple suppliers. The absence of a single specialized chip can bring the manufacture of end products to a halt, even if all other components are available.
The production process is also more complex and multilayered than headline writers often choose to acknowledge. When all production phases are considered, the entire process extends from material procurement to back-end manufacturing (Exhibit 1). (Some may choose to take a narrower view, in which the value chain begins at the design phase.) For each product segment, most companies specialize in three or fewer steps and may outsource some activities, such as printed-circuit-board assembly, to partners. After back-end manufacturing, semiconductors become part of the electronics value chain.
The industry has become increasingly consolidated within many of these value chain segments over the past 20 years, and a few champions have emerged in each area (Exhibit 2). As a result, expertise is often concentrated in certain markets (for instance, the United States has the highest presence of fabless players—that is, chip design only—and equipment manufacture). No local market has all the capabilities required for end-to-end semiconductor design and manufacturing, and the concentration of expertise has created a web of interdependencies along the value chain (Exhibit 3).
The concentration of expertise conveys some advantages because it often allows companies to share resources, such as power supply, even if they are rivals, which helps keep costs down. Employees with the right skills may also gravitate to expertise clusters, creating a strong talent pool. But the interdependencies also mean that local shocks can have a global effect, such as when a flood in Thailand in 2011 stopped production in multiple memory backend fabs in the country and pushed prices of memory chips 30 percent higher.
Factors behind the semiconductor shortage
Semiconductor fabs were already operating at close to full capacity before the pandemic because they try to avoid investing in new capital equipment beyond that required to meet demand from customers. Uncertain trade dynamics also motivated several players to increase their semiconductor stock levels to secure supply. The pandemic, which spurred purchases of computers and other devices for remote work, then took demand to even greater heights.
For automotive OEMs and other companies, one response has been to deviate from their typical “just in time” ordering. Instead, they have started to order more chips than necessary so that they can build their inventory and have reserves on hand. In the short term, however, this move has magnified the gap between supply and demand. Over the longer term, some companies may consider requesting binding “take or pay” contracts in which they can either accept a certain quantity of chips or pay a fee if they decline to do so. This arrangement helps companies align chip demand with manufacturing capacity more accurately.
Customers may also consider coinvesting with semiconductor companies in projects designed to increase fab capacity. Such projects can allow semiconductor companies to reduce their up-front investment, relieving potential capital expenditure constraints. But coinvesting will not immediately improve the semiconductor shortage, given the long timelines for fab construction and production ramp-up.
Achieving and maintaining a leading position in the semiconductor industry
Despite the current uncertainty, the semiconductor industry is poised for additional growth, as more and more products and services become increasingly digitized. More than half of the semiconductor industry’s current enterprise value is based on earnings-growth expectations, as is reflected in current valuations: investors expect long-term growth of 7 to 8 percent per annum, assuming the recent margin trajectory.
But what strategies can help the industry meet these targets? While the answer may vary based on a company’s strengths and weaknesses, all semiconductor companies could benefit by rethinking their approach in six critical areas: technology leadership, long-term R&D, resilience, talent, ecosystem capabilities, and greater capacity. The final area here will not provide immediate benefits, of course, but it could be an important part of a long-term strategy. We have quantified the costs associated with fabs of different sizes to help semiconductor companies determine if capacity expansion is right for them.
Based on a recent analysis of integrated device manufacturers (IDMs) and fabless players, we believe that strong growth is possible for all semiconductor companies, regardless of size (Exhibit 4). Although the largest companies generated the greatest economic profit, there are also small, niche players with high operating margins.
Technology leadership
Across all product segments, semiconductor companies strive for innovation because faster, more powerful chips and leading-edge equipment help generate greater sales in all value chain segments. The companies with the most distinctive technologies and products are likely to become the global champions. In a cross-industry analysis, the semiconductor sector was second only to pharmaceuticals and biotechnology for R&D spending—calculated as a percent of sales (Exhibit 5). When we examined industry champions, we found that they often succeeded by incorporating the following strategies into their R&D plans.
A focus on leading-edge chips and the machinery required to create them. For semiconductor manufacturers, creating smaller node sizes has traditionally been the path to success. For decades, the number of transistors on a chip doubled every two years—the rate predicted by Moore’s law—as semiconductor companies constantly decreased the size of technology nodes. In recent years, however, the rate of doubling has slowed because technological challenges increase as the industry approaches the physical limits for the number of transistors that can be included on a single chip. Nevertheless, companies will still attempt to push the technology, because average demand growth for chips with the smallest nodes—seven nanometers (nm) and below—will be four percentage points higher than supply growth through 2025.
The importance of node size varies by device segment, and the demand for leading-edge chips will grow much more in some categories than in others. Since customers expect high performance for compute-intensive applications, semiconductor companies that design chips in the smallest available technology node may have a distinct advantage in these areas. In other segments, larger nodes are often suitable because customers are satisfied with current chip performance or require specific features, such as fast switching, and see little advantage in moving to smaller node sizes.
Equipment manufacturers may capture growth by creating the machinery required to enable leading-edge innovations. In addition, they could create equipment that includes advanced technologies to optimize process control, as well as yield monitoring and enhancement.
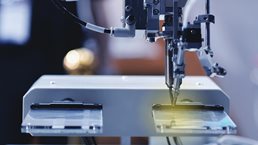
Value creation: How can the semiconductor industry keep outperforming?
Demand for specific mature nodes (40 to 65 nm) is also higher than average, since they are used in automobiles and other critical products. Their profit margins historically tend to be lower, however, making it sometimes difficult to support a business case for expanding mature-node capacity. If new fabs are built, the high up-front costs will mean that companies will initially see lower profits from them than they will from existing fabs with depreciated assets. To ensure solid returns over the long term, players could work to get hard commitments from their customers to guarantee that new fabs will have high utilization once on line.
Differentiation through ‘more than Moore.’ Beyond reducing structure size, some semiconductor companies are pursuing “more than Moore” innovations to make their products distinctive. Some, for instance, are developing semiconductors based on materials other than silicon. Compound semiconductor materials, such as silicon carbide (SiC) and gallium nitride (GaN), are particularly well suited for applications requiring both high power and frequency, since they limit energy loss and allow for the creation of smaller form factors.
The push for increased sustainability and electrification is spurring the adoption of SiC and GaN power devices, and the compound annual growth rate (CAGR) for both categories is expected to far exceed the 5 percent growth forecasted for the power semiconductor market as a whole (Exhibit 6). In the base-case scenario, annual market growth is expected to be 23 percent for SiC devices and 40 percent for GaN power devices.
Equipment manufacturers could promote innovation and capture new opportunities by launching machinery specialized for processing SiC or GaN. Given that demands for these machines will be lower than that for mainstream leading-edge equipment, manufacturers should carefully review the business case for developing these initially niche products. Foundries could provide access to SiC- and GaN-based innovation to a broader base of fabless semiconductor players.
A focus on innovative features may be particularly valuable in high-growth segments, such as the Internet of Things, because it helps differentiate products from competitors (for instance, by optimizing the fast switching required in several radio frequency applications). Several IDMs and foundries are already developing such products in mature nodes.
Advanced packaging of semiconductor components. These techniques provide better heat management during operations, allowing companies to place semiconductor components closer together. With a greater number of connection points, these chips provide higher data-transfer rates and better performance. In addition, advanced packaging allows semiconductor companies to combine mature and leading-edge chips in an integrated system for applications that need both types, which lowers costs. This trend, called heterogeneous integration, enables companies to combine multiple smaller chips instead of making one large chip. Larger chips often have lower yield, with the drop typically scaling with chip size, so heterogeneous integration may deliver profound cost benefits.
The advanced-packaging market was valued at $20 billion in 2020, and this figure is expected to rise to $45 billion by 2026, when it will represent about 50 percent of packaging revenues. Although leading-edge IDMs and foundries are driving packaging innovations, advanced techniques also create opportunities for other players across the value chain because they boost demand for new materials and new equipment.
Specialized applications. Application-specific integrated chips (ASIC) integrate well-defined algorithms and functions into their chip design and are customized for specific purposes, such as for use in artificial intelligence and cloud computing. The segment has recently grown significantly and could offer a good opportunity for additional players. Small companies that want to focus on developing specialized semiconductors could still find their products in high demand, even if their customer base is relatively small.
The customer base for ASICs includes many different companies, such as automotive OEMs and hyperscalers, and their needs will vary. Some customers may decide to design their own ASICs in-house to improve customization, differentiate their products, and reduce lead times. They would then work directly with foundries for their manufacturing needs. Other players, typically smaller ones, prefer to have an ASIC partner that is responsible for all steps needed to move from design to end product because this requires many specialized competencies.
Bold, long-term R&D investments
R&D cycles within the semiconductor industry can be very long, sometimes extending beyond ten years, and companies typically do not see immediate returns. Historically, some governments have helped fund such work, since most publicly listed companies do not always have the appetite for such long-term investments.
Although many investors may be skeptical of providing funding over an extended period, semiconductor companies have proven that bold, long-term investments can eventually deliver substantial returns. For instance, ASML spent 17 years and about $7 billion developing its extreme-ultraviolet lithography technology, including the ability to produce the technology at volume. The long R&D timeline was worthwhile, since the tool is now a major source of revenue for ASML. Similarly, Arm spent six years developing a 64-bit computing processor that is now a significant source of the company’s revenue.
Other companies that invest heavily in long-term R&D projects could help promote technological leaps—often far more than node reduction—that could help improve society. The creation of specialized chips for quantum computing, for example, could improve pharmaceutical development, sustainability programs, and other initiatives across industries. In most cases, semiconductor companies are focusing on increasing investment in areas where they are already strong, rather than branching into new areas to extend their technological advantage even further.
Greater resilience in a volatile world
Like other businesses, semiconductor companies and other industry stakeholders are still attempting to develop new strategies to manage the huge disruptions arising from the COVID-19 crisis, including supply chain problems and shifting demand. One thing is clear: the postpandemic world will likely remain more volatile, which will require greater resilience from companies.
In markets with a large mismatch between local supply and demand, semiconductor companies could consider dedicating more capacity for the nodes that are most urgently needed to provide some short-term relief. For instance, European end customers largely require semiconductors with nodes larger than 28 nm for automotive and industrial applications, while customers in the United States have a much higher need for semiconductors with nodes smaller than seven nm. Additional capacity might best be tailored to meet such needs. In addition to helping the local market, these moves would increase supply chain resilience and lessen intermarket dependencies.
Semiconductor companies could also help decrease lead times by becoming more agile and responsive, such as by expanding their supplier base, strengthening pricing strategies, improving chip allocation to customers, working with industry organizations to explore solutions to the chip shortage, and inviting customers to coinvest in the development of custom chips.
The current economic situation calls for increased agility. While demand for semiconductors is strong now, a downturn in major markets—always a possibility—could necessitate new strategies to minimize capital expenditures and maximize revenues. For instance, semiconductor companies might consider revising their down-payment policies and charge customers up front for dedicated capacity, or they might require customers to provide binding demand forecasts more than 18 months in advance.
A strong talent pipeline
As semiconductors become more critical to product differentiation, some electronics companies, automotive OEMs, and hyperscalers are moving chip design in-house to increase customization and eliminate bottlenecks. These moves are making competition for already-scarce semiconductor talent even tougher than usual. Simultaneously, chip design is becoming more and more complex as the functions of semiconductors expand, which requires more labor. The increase in labor is especially high with five-nm nodes, which are the most difficult to design and require the most days of labor (Exhibit 7).
With greater competition, semiconductor companies would be wise to increase their efforts to recruit talent, including staff with expertise in process technology and operations management. But first, they must address an image problem. In a recent survey of employees, the semiconductor industry ranked lower than tech and automotive on multiple dimensions of workplace attractiveness, including work–life balance, career opportunities, and diversity and inclusion (Exhibit 8). Companies may want to review their own operations to ensure that they are competitive in these areas.
As semiconductor companies step up recruitment, they may need to build their brand. Typically, semiconductor products receive less attention than end products, and prospective employees may have little knowledge of the many strong and innovative companies within the sector. Companies might also need to review compensation and learning and development opportunities to ensure that they are on par with businesses in other industries.
One possible solution to the labor shortage might involve forming partnerships with academic institutions, especially in markets where talent is in very short supply. If semiconductor companies consider supporting learning programs, and perhaps provide guidance on classes offered, graduates are more likely to have the skills required to work in the industry.
Improved collaboration within the semiconductor ecosystem
The increased complexity of chip design, combined with shifts in the value chain and greater competition for talent, have increased the importance of ecosystem building within the semiconductor industry. Partnerships with customers are already becoming more common. For instance, many automotive OEMs that want to improve their design capabilities are now collaborating with semiconductor companies, especially on the development of application-specific solutions, such as those for autonomous vehicles.
In another type of collaboration, companies may create ecosystems in which one player develops intellectual-property (IP) blocks that many customers can leverage. Arm, for instance, has developed an architecture for a processor that others may license. This strategy decreases costs for all involved. Some companies have also formed strong IP partnerships with academic institutions.
Major semiconductor players also have a long history of joining forces to develop and align their technology blocks, thereby reducing the chance that one organization will create a technology that does not fit into the value chain. Similarly, industry associations can play an important role in providing guidance on the long-term technology road maps, and dedicated semiconductor research institutes, such as Imec, in Belgium, may convene players to collaborate during precompetitive research.
Beyond partnerships, semiconductor companies might want to undertake a programmatic M&A strategy—a serial approach to smaller acquisitions, along a specific theme—as the industry consolidates. If they do so, they might benefit by focusing on acquisitions that would allow them to branch into adjacent areas, open important markets, or add capabilities essential for future growth and for extending their technology leadership. The current scarcity of targets, however, requires potential acquirers to investigate and execute mergers quickly.
Collaboration with other ecosystem members could also take more informal shapes. If multiple semiconductor companies agree to build or expand hubs for design and manufacture, they could have an easier time attracting talent and might obtain other benefits, such as the ability to collaborate on IP development.
Greater capacity
For some semiconductor companies, capacity expansion could deliver benefits. But given the enormous sums required for construction and equipment, company leaders must carefully consider plant capacity before moving ahead. Operating and construction costs, when indexed per 12-inch mask layer, fall as capacity increases. For instance, indexed construction and operating costs are both around $3 billion for fabs with a capacity of 250,000 layer starts per week (LSPW). Indexed construction costs drop to between $2 billion and $3 billion for fabs with a capacity of 400,000 LSPW, after which they plateau. Operating costs reach a plateau for fabs with a capacity of around 575,000 LSPW.
Our analysis suggests that fabs with a capacity of at least 600,000 LSPW generate the best cost position (Exhibit 9). This would correspond to 12,000 to 20,000 wafer starts per week, depending on the product. The type of chip being produced will significantly affect costs, which increase exponentially as node size shrinks. Building a 40-nm fabrication plant with a capacity of at least 600,000 LSPW would likely require around $5 billion, with about 80 percent of the investment going to equipment expenses. But leading-edge fabs producing the smallest node sizes might cost $10 billion or more.
Semiconductor companies might be able to obtain some cost advantages by building fabs in areas where there is already a cluster of similar businesses, since this may help ensure sufficient talent availability and resources (such as land, energy, and water). In another cost-saving move, players could attempt to improve tool hookup and ramp-up to earn back the cost of their investment more quickly. Their efforts might involve forming partnerships with equipment manufacturers to apply advanced analytics that increase yield. For example, modeling made possible by advanced combinatorial learning could potentially replace some elements of physical testing throughout the full manufacturing cycle, decreasing both costs and time to market. For instance, companies could switch from physical metrology to virtual metrology using data.
Some government officials in markets where chip demand outstrips local supply are also thinking about strategies to increase local front-end manufacturing capabilities, particularly since greater supply chain disruptions and geopolitical issues have recently complicated trade. In some cases, they may subsidize fab construction, which research suggests can help companies recoup their investment more quickly. But many government officials may not be aware of all the challenges they will face in trying to catch up to leading markets. (For more information on these topics, see sidebar, “Should local markets increase foundry capacity and other semiconductor capabilities?”)
Since the semiconductor value chain is so complex and specialized, the industry will likely remain a highly interdependent global network for the foreseeable future. And as no market has companies with all the capabilities needed along the entire end-to-end value chain, each one should focus on strengthening its position in areas where it now leads. This strategy will help markets stay relevant as the latest era of semiconductors
While they may be hidden in devices, semiconductors now play a more critical role than ever in the lives of everyone from schoolchildren to patients in nursing homes. The current semiconductor shortage has highlighted this fact and has made the industry’s dependency on a well-functioning semiconductor supply chain very visible. Some of the most exciting trends, including those related to autonomous driving, vehicle electrification, and artificial intelligence, rely on ongoing innovation in semiconductor technology and a stable chip supply. We believe these trends could turn the next ten years into “the golden semiconductor decade”—if stakeholders prepare now to capture the opportunities ahead.